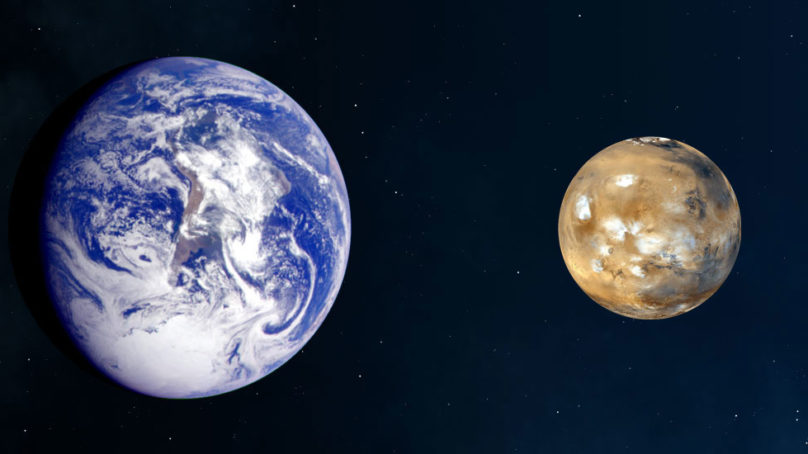
Astronomers armed with optical telescopes fell in love with Mars during the very earliest years of the age of telescopes. Mars was bright, changed colors, and had intriguing, contrasting brighter and darker regions. Furthermore, because Mars is closer to Earth than all other planets except Venus, it could appear quite large when viewed in a telescope. Mars lured astronomers in and kept their attention. By the end of the eighteenth century, knowledge gained by astronomers about the physical properties of Mars would convince them that because the two planets shared so many properties, we could think of them as twins.
Almost as soon as telescopes became common, observations of Mars took center stage. Father Daniello Bartoli, a Jesuit observing with his telescope on Christmas Eve, 1644, detected two patches on the “lower part” of Mars. He recorded no other observations of Mars, but did preserve for us one other personal observation. “God willing,” he wrote, “future observers might be able to see them better.”
In the late 1650s, because of the confluence of the fortuitously good positioning of Mars for observations and the arrival on the scene of one of the most brilliant astronomers of the century, Christiaan Huygens of the Netherlands, a Martian revolution was about to occur. During the early evenings of November 28 and December 1, 1659, Huygens drew sketches of Mars that show a large, broad, dark “V”-shaped area that covered about half the breadth and half the height of the visible disk of Mars. Based on the difference in times between his observations on the two evenings and the slight shift in position of the dark patch from the night of November 28 to the night of December 1, Huygens drew a bold and correct conclusion: the rotation of Mars, like that of Earth, has a period of about 24 hours.
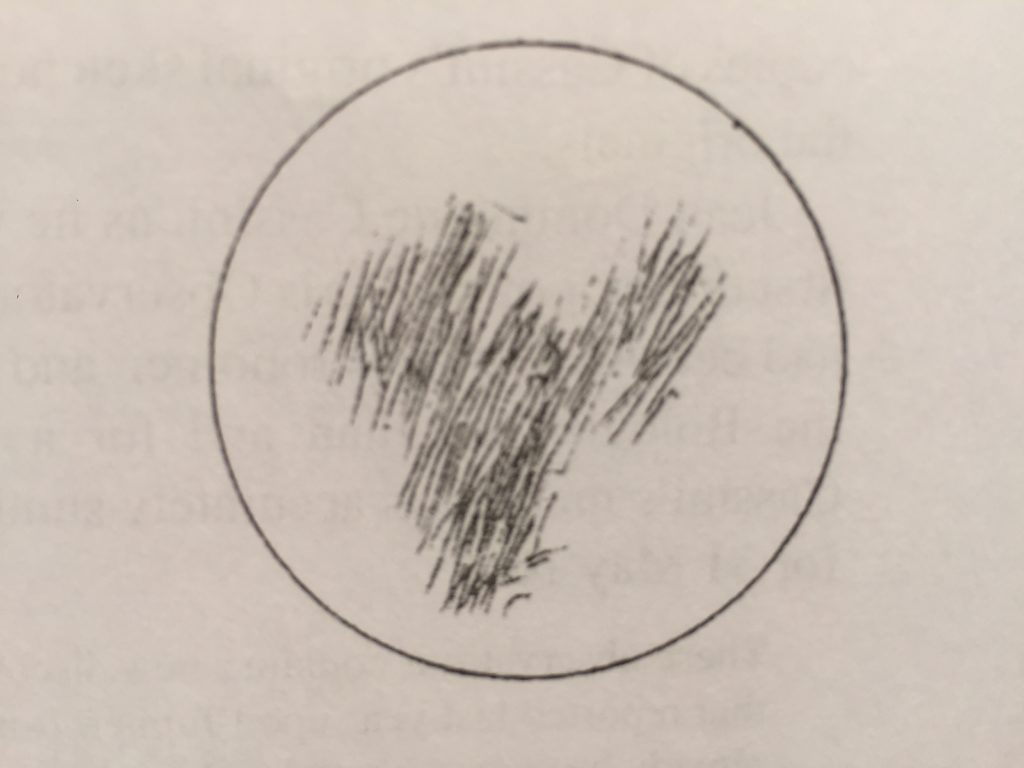
Sketch of Mars made by Christiaan Huygens, made on November 28, 1659. The dark patch in this sketch, first seen by Huygens and one of the most easily identified features on the surface of Mars, is now known as Syrtis Major Planum. In the nineteenth century, it was variously known as the Hourglass Sea (or Mer du Sablier), the Atlantic Canale and the Kaiser Sea.
Image source: Flammarion, La Planète Mars, 1892
Let that discovery marinate a moment. Mars rotates; Mars has a day and night; and the length of the cycle of day and night on Mars is nearly identical to the 24-hour cycle of day and night on Earth.
The rotation period of Mars did not have to be 24 hours. After all, the rotation periods of planets in our solar system range from a few hours to hundreds of days. Why 24 hours for Mars? For astronomers in the seventeenth century, the answer was obvious: Mars is Earthlike. With this discovery, the appeal of Mars as the important planet for study mushroomed.
In 1666, Italian and papal astronomer Giovanni Domenico Cassini carried out a series of observations of Mars. Not only did Cassini see two dark patches on Mars, he observed them as they moved from east to west across the observable disk of the planet, day after day. Curiously, these dark patches did not return to their same positions exactly 24 hours later. Instead, they reached the same position 24 hours and 40 minutes later. Mars, he concluded correctly, completes one turn on its axis in 24h 40m, rather than exactly 24 hours.
The next great advances in our knowledge of Mars—and in making Mars seem even more Earthlike—emerged from the observational work done by Giacomo Filippo Maraldi, the nephew of the great Cassini. Cassini hired Maraldi as an assistant astronomer at the University of Paris Observatory, where his nephew then spent his career making observations of planets. Maraldi made four major discoveries during his studies of Mars in 1704, during the closest opposition of Mars that had occurred in 15 years. Then, after patiently waiting another 15 years, he confirmed these results during the next close opposition in 1719. First, Maraldi ever so slightly improved our knowledge of the rotation period of Mars. Mars, he determined, rotates in 24h 39m rather than 24h 40m. He also established that Mars has dark patches and that, unlike the dark patches on Earth’s Moon, the Martian dark patches vary in both form and location.
Finally, Maraldi discovered that Mars has bright patches at the north and south poles that changed in appearance over time. In fact, the bright patch in the south, which was offset slightly from the exact southern pole, sometimes disappeared entirely, as did one bright patch in the north. Maraldi took great care to avoid trying to explain the bright polar spots, though he did conclude that the changes in appearance were due to some genuine physical change on the surface of Mars. Not surprisingly, astronomers of that era did not need much imagination to surmise that these bright patches were polar ice caps, similar to those on Earth.
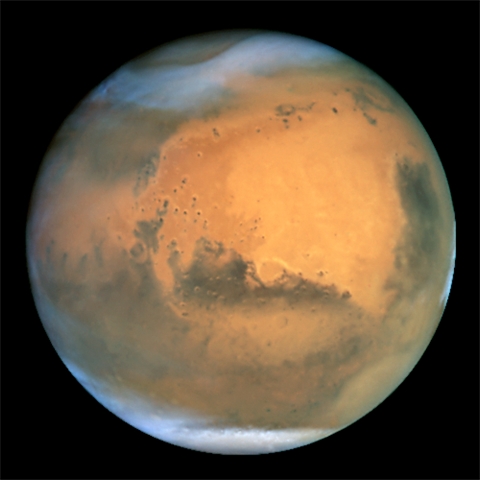
Hubble Space Telescope image of Mars, as seen in 2001 at a distance of 43 million miles (68 million kilometers) from Earth. Ice is evident at the southern polar cap (bottom) while a dust storm obscures the northern polar cap (top). A second giant dust storm can be seen in the Hellas Basin (lower right). Water ice clouds are seen surrounding the north polar cap, extending northwards from the south polar cap, and near the Martian equator.
Image courtesy of NASA and The Hubble Heritage Team (STScI/AURA)
Further progress in our knowledge about Mars was slow through most of the eighteenth century, but exploded again with the work of William Herschel, in the 1780s. Herschel’s list of important scientific accomplishments, almost all of which he accomplished with the dedicated help of his sister Caroline, is long. He discovered the planet Uranus. He proved that some stars orbit other stars in so-called binary star systems. Herschel also made a map of the entire sky, noting the location and apparent distance of every star he could see. Thinking that the faintest visible stars in the heavens marked the distant edge of the universe, he thought that he had mapped out the entire universe. Herschel’s Universe turns out to be a map of part of our galaxy, the Milky Way, not of the entire universe, though astronomers would not understand that until the 1920s, when Edwin Hubble revolutionized astronomy with his work. Herschel also discovered the existence of light outside of the range at which our eyes can see; he found light beyond the red that we now call infrared light. That extensive but not exhaustive list of important discoveries and measurements justifies his historical position as the greatest astronomer of the eighteenth century.
With regard to Mars, Herschel made a number of very careful observations, one of which took astronomers of his age one step farther down the road in turning Mars into a clone of Earth. Herschel discovered that the sizes of the bright northern and southern polar spots, the presumed ice caps first seen by Maraldi a century earlier, waxed and waned anti-synchronously. When the northern polar spot shrinks, the southern polar spot grows. When the northern polar spot expands, the southern polar spot contracts. That pattern of behavior, he proposed, is due to seasonal changes in the ice caps.
From his careful and extensive observations made across the years from 1777 through 1783, Herschel was able to prove that the rotation axis of Mars is tilted relative to the plane of its orbit around the Sun (astronomers call this property of a planet the obliquity) by 28.7˚, and he measured the rotation period to be 24h 39m 21.67s. He was just a bit off on both measured values. The obliquity is closer to about 25.2˚ and the rotation period is closer to 24h 39m 35s. But we should give him credit for work well done. More importantly, the 25˚ obliquity of Mars is nearly identical to the 23.5˚ tilt of Earth’s rotation axis relative to Earth’s orbital plane around the Sun. This tilt for Earth, and not the changing distance of Earth to the Sun, is the primary reason for the existence of seasonal changes on Earth. Thus, Mars, with an obliquity nearly identical to that of Earth, also must have spring, summer, fall, and winter, and those seasons will occur at opposite times in the northern and southern Martian hemispheres, just as they do on Earth, where summertime in Australia occurs during wintertime in Alaska. Herschel’s discovery of the obliquity of Mars proved, almost certainly, that Mars’s bright polar spots were ice caps. (Exactly what kind of ice would be debated for another century.)
Mars was becoming more and more Earthlike with every new discovery. Herschel concluded, in his Second Memoir, read to the Bath Philosophical Society in England on March 11, 1784:
The analogy between Mars and Earth is certainly more evident than for any other planets in the Solar System. Their diurnal movement [the length of the day] is almost the same; the obliquity of the ecliptic, causing the seasons, is analogous; of all the superior planets [those further from the Sun than Earth], the distance of Mars from the Sun most nearly resembles that of Earth, and as a result, the length of the Martian year is not enormously different from ours.
Finally, Herschel concluded that Mars has an atmosphere. On the one hand, because of the variations in brightness in the planet in certain regions that he attributed to the presence of clouds and vapors in the atmosphere, he concluded that the atmosphere was considerable. On the other hand, he could observe stars that appeared as close as 3 to 4 minutes of arc (between 1/20th and 1/15th the angular diameter of the full moon) to the limb of the planet that did not change at all in brightness as they moved closer to Mars. From these observations, he concluded that the Martian atmosphere did not extend very far from the surface; otherwise the Martian atmosphere would have blurred and extinguished the light of these stars when Mars passed so near to them.
The last major contributions to our knowledge of Mars that occurred before a group of nineteenth-century astronomers would begin mapping the surface of Mars were made by the accomplished German astronomer Johann Hieronymus Schröter, who had his own observatory in the city of Lilienthal, where he was the chief magistrate.
Schröter observed Mars almost continuously for 18 years, from 1785 through 1803, producing 230 different drawings of it. He confirmed most of Herschel’s discoveries, getting similar but slightly different values for the obliquity (27.95˚) and the rotation period (24h 39m 50s). Schröter’s most important new contributions to our knowledge of Mars were his observations of continual changes, sometimes hourly, in the patterns of the dark patches on Mars. These patterns were never the same, from night to night and year to year. Schröter concluded that clouds were responsible for the changing colors he observed on Mars; in fact, he came to believe that the patches themselves were entirely atmospheric phenomena rather than surface features.
By the time the eighteenth century ended, two centuries of telescopic observations of Mars that had begun in the early 1600s had yielded a reasonable portrait of the red planet. Astronomers had accurately measured the rotation period, the axial tilt, the existence of polar caps that waxed and waned with the seasons, and the presence of a thin atmosphere with clouds that could, at times, obscure parts of the surface. What they concluded was self-evident to astronomers and to anyone else paying attention: the rotation period of Mars is just like the day/night spin of Earth, the obliquity of Mars is just like the tilt of Earth, the seasonal changes of Mars are just like the seasons we find here on Earth, the polar caps on Mars are just like the ice caps on Earth, and the thin atmosphere that is sometimes transparent and at other times is opaque with clouds behaves just like the cloudy atmosphere of Earth. Mars and Earth, they had established, are physical twins.
About the Author
David Weintraub is Professor, Director of Undergraduate Studies and Director, Program in Communication of Science & Technology at The Department of Physics and Astronomy at Vanderbilt University. His new book, Life on Mars: What to Know Before We Go takes a step back from the all-systems-go approach to colonizing another planet and considers the ethics of potentially destroying its bio-ecosystem. There’s no better time to think about the implications of such a trip, Weintraub contends, with both NASA and entrepreneur Elon Musk working to make it happen. Weintraub is also the author of Religions and Extraterrestrial Life: How Will We Deal With It? and two other books.
This story is excerpted from LIFE ON MARS: What To Know Before We Go by David A. Weintraub. Copyright © 2018 by Princeton University Press. Reprinted by permission.