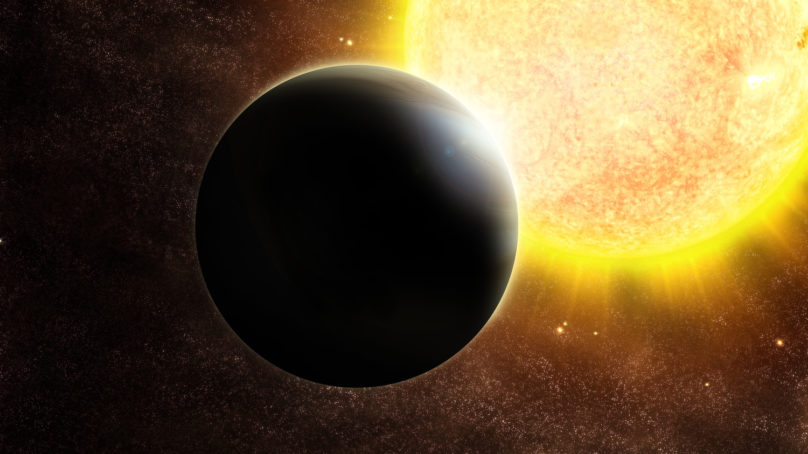
Do there exist many worlds, or is there but a single world? This is one of the most noble and exalted questions in the study of Nature. — St. Albertus Magnus (1206–1280)
Take a look at the image in Figure 1. It may not look like much, but the four labeled blips of light come from four planets orbiting a star that lies far beyond our own solar system. This image is therefore part of an incredible scientific revolution that is now underway, and that is helping us understand the answer to the exalted question (above) raised by St. Albertus Magnus some 800 years ago.
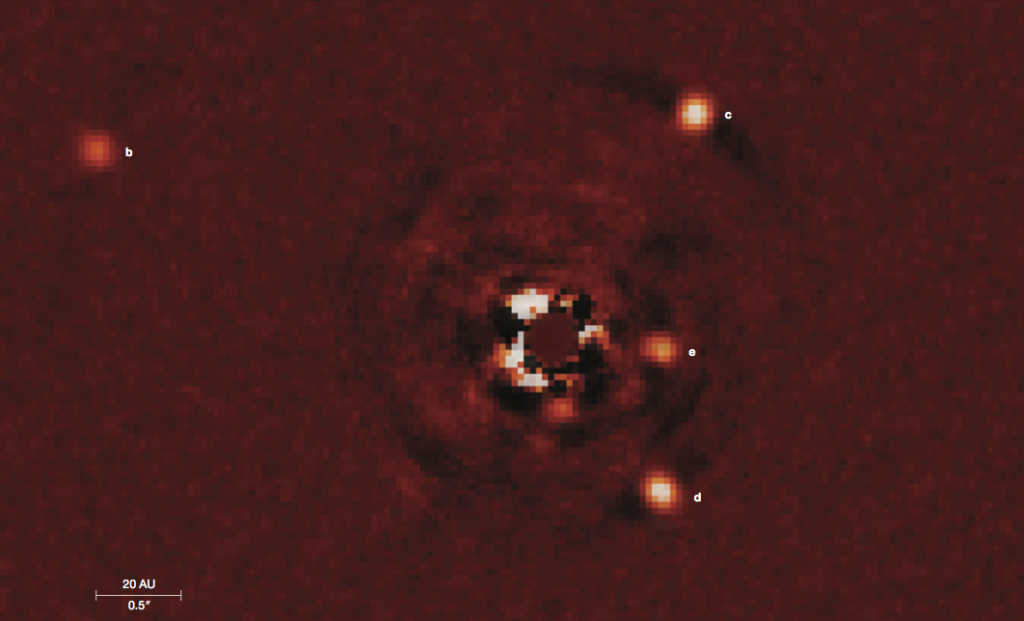
Figure 1. This infrared image shows four planets (labeled b, c, d, e) that orbit the star HR 8799. Light from the star itself (center) was mostly blocked out during the exposure, as indicated by the solid red circle. Click the image above to see a time-lapse video showing the orbital motion of the planets. Credit: A.-L. Maire / LBTO.
St. Albert raised the question at a time when Earth was still generally assumed to be the center of the universe. We first learned otherwise about 400 years ago, when scientific evidence collected during the Copernican revolution left no reasonable doubt that Earth is a planet orbiting the sun. This fact opened the possibility that the stars might be other suns with their own planets. Still, as recently as the early 1990s, we did not know for sure whether any such extrasolar planets, or exoplanets for short, really existed. Today we know of thousands, and the number is rapidly growing. Indeed, it now seems likely that many — and perhaps even most — of the hundreds of billions of stars in our galaxy have their own planetary systems, and the same is presumably also true among the more than 100 billion other galaxies in our observable universe. All in all, there are probably as many planets (and stars) in the universe as there are grains of sand on all the beaches of Earth combined. Imagine what St. Albert would think now.
The scientific revolution of exoplanets is remarkable on many levels, starting with the technological one. To understand the challenge involved, consider what our solar system looks like on a scale of 1-to-10 billion, as it does in the Voyage scale model shown in Figure 2. On this scale, the Sun is about the size of a large grapefruit, while the largest planet, Jupiter, is only about the size of a marble and Earth is smaller than the ballpoint in a pen. Yet while you can walk from the Sun to Pluto in just a few minutes on this scale, you’d need to continue the distance across the United States to reach even the nearest other stars. In other words, detecting exoplanets is rather like trying to see ball points or marbles more than 4000 kilometers away, a problem further compounded by the fact that the tiny planets shine only with light reflected by a star that is typically a billion times brighter than they are.
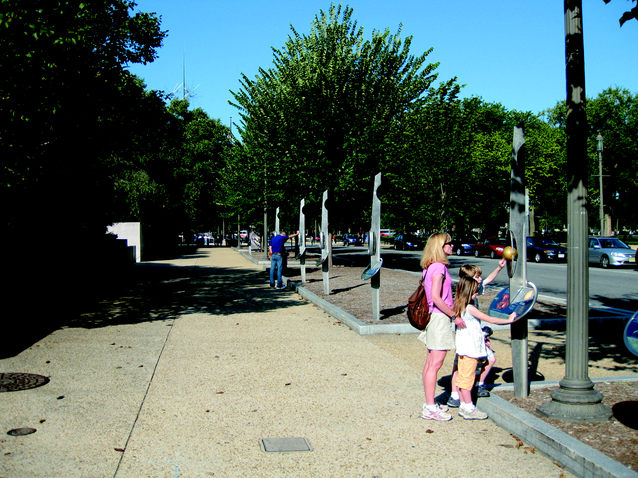
Figure 2. This photo shows the pedestals housing the Sun (gold sphere on nearest pedestal) and the inner planets in the Voyage Scale Model Solar System (Washington, DC). The model planets are encased in the sidewalk-facing disks visible at about eye level on the planet pedestals. To the left is the National Air and Space Museum. Click the image above to learn how your community can get its own Voyage model.
Given the observational challenge, you probably won’t be surprised to learn that we have relatively few actual images of planets like those in Figure 1. Instead, most of the thousands of known exoplanets have been identified through indirect methods, which fall into two major categories.
The first category involves looking for small motions of a star caused by the gravitational tug of orbiting planets. Two major methods can detect these tugs. The astrometric method seeks to observe the small changes that will occur in a star’s position in the sky. This method has had limited success to date, but the European GAIA mission (launched in 2013) is expected to change that over the next few years as it continues to measure stellar positions with unprecedented precision. The greater success to date, which includes most of the first several hundred exoplanet discoveries, has come with the Doppler method, which relies on the same idea used by radar guns to catch speeders: an object moving toward you will have the wavelengths of its light (or of reflected radio waves used in radar) compressed to shorter wavelengths (a blueshift) and an object moving away from you will have its light stretched to longer wavelengths (a redshift). As shown in Figure 3, scientists can therefore recognize the existence of orbiting planets by detecting alternating redshifts and blueshifts in the spectra of distant stars.
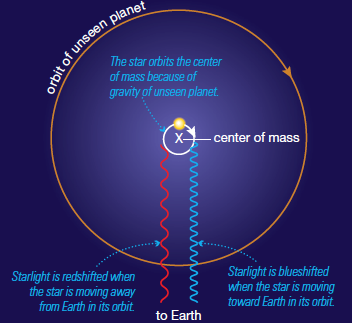
Figure 3. The gravity of an unseen, orbiting planet (outer circle) causes its star to trace a small orbit (inner circle) around their mutual center of mass. As a result, if the system is oriented so that the star moves alternately toward and away from Earth, we can in principle detect alternating redshifts and blueshifts in its spectrum. Credit: The Essential Cosmic Perspective, 8th Edition, by Bennett, Donahue, Schneider, and Voit (Pearson, 2017).
The second general approach to indirect detection seeks to measure changes in a star’s brightness as one or more orbiting planets pass in front of it as seen from Earth. This is done through what we call the transit method, which works only when a planet happens to orbit edge-on as seen from Earth. In that case, as shown in Figure 4, the star’s light will dim slightly during the time that the planet passes in front of the star as viewed from Earth. The transit method is the current champion of planet discoveries, thanks to the Kepler spacecraft, which used this method to find evidence for some 4,000 planets orbiting some 2,000 stars during its main observing mission from 2009 to 2013.
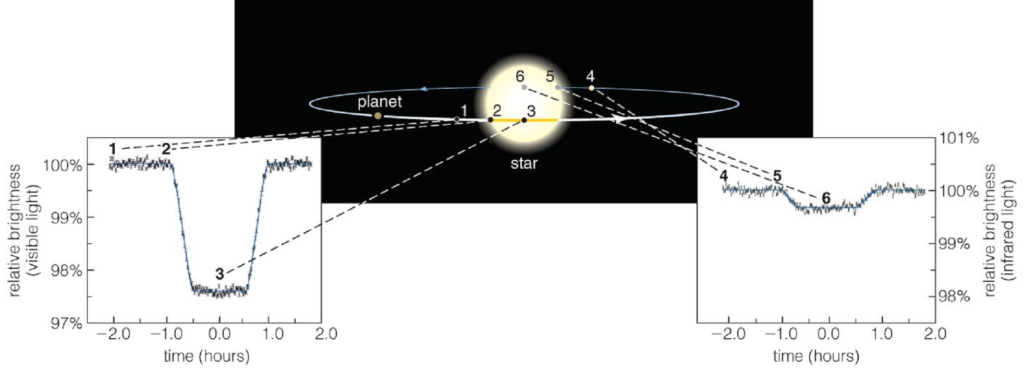
Figure 4. The central diagram shows a planet orbiting a star edge-on as seen from Earth. The left graph shows the dimming of starlight that occurs each time the planet passes in front of the star; the right graph shows that there is also infrared dimming when the star passes behind the star, since the planet contributes to the system’s infrared brightness. The data are for a star called HD 189733. Image credit: The Essential Cosmic Perspective, 8th Edition, by Bennett, Donahue, Schneider, and Voit (Pearson, 2017).
In addition to telling us that a planet exists, these indirect methods can provide us with a surprising amount of information about the planet. For example, all of the methods can tell us a planet’s orbital period and distance from its star, while the Doppler and astrometric methods can help us learn the planet’s mass and the transit method can tell us a planet’s size. When we have both size and mass information, we can calculate a planet’s average density. Table 1 summarizes the major ways in which our planet detection methods help us learn about exoplanets.
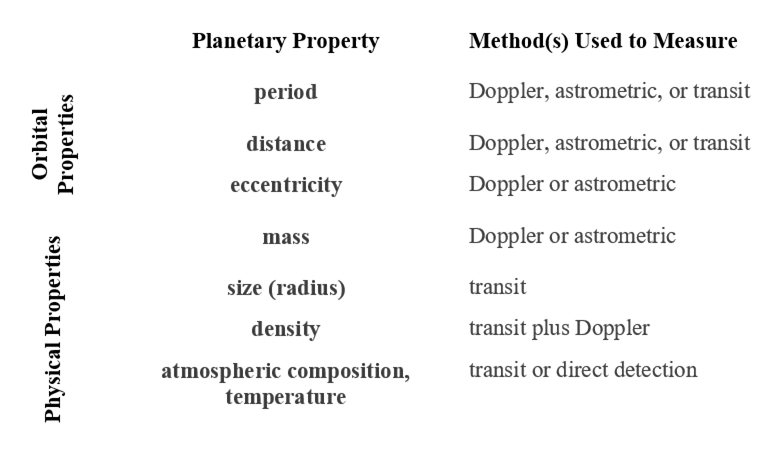
Table 1. A summary of the major ways in which we can learn properties of exoplanets.
By combining these physical data with model-based inferences, scientists can make predictions about the nature of different exoplanets. This idea can be neatly summarized with a “planetary mass-radius diagram” (or “M-R diagram”), as shown in Figure 5. (Readers familiar with stellar astronomy will recognize similarities between the ways in which scientists can use this M-R diagram for planets and the ways that astronomers have traditionally used the more famous H–R diagram (Hertzsprung-Russell diagram) for stars.) Because mass and radius together yield density, the graph also shows selected density curves (dashed lines).
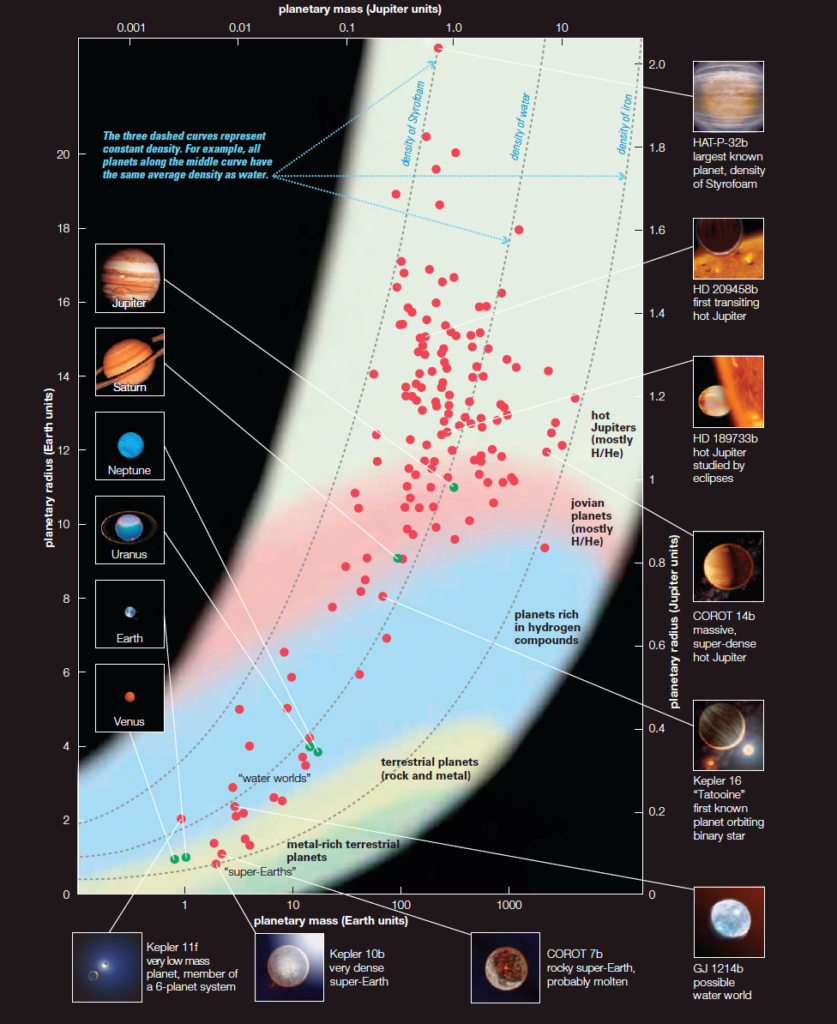
Figure 5. This “planetary mass-radius diagram” show a sample of exoplanets for which both mass and radius have been measured (red dots), along with the planets of our own solar system (green dots). Image credit: The Cosmic Perspective, 8th Edition, by Bennett, Donahue, Schneider, and Voit (Pearson, 2017). Paintings by Michael Carroll.
The planetary mass-radius diagram offers a wealth of information, but perhaps its most important lesson can be seen simply by noticing the difference in scatter among the green dots for the planets of our own solar system in comparison to the red dots for exoplanets. Clearly, planets come in a far wider range of types than we could have known from studying only the planets that orbit our sun. For example, notice the labels indicating regions of the diagram that represent “hot jupiters” (Jupiter-like planets that orbit very close to their stars), “water worlds” (planets with compositions dominated by water or other hydrogen compounds, though not necessarily in liquid form), and “super-Earths” (planets with Earth-like composition that are larger or more massive than Earth).
In addition to learning about planetary nature, current data allow scientists to make some statistical statements about how many stars have planets. The Kepler mission again holds the key to date, because it made its planetary discoveries while monitoring a known number of stars. Therefore, based on the mission’s technological capabilities, scientists can use the Kepler data to calculate approximate minimums for the percentages of stars that have planets of various sizes.
Figure 6 shows the results, and they are quite remarkable. For example, the first bar shows that at least about 17% of stars have one or more planets similar in size to Earth. Keep in mind that these percentages are almost certainly underestimates that will rise as new technological capabilities enable us to detect planets that are undetectable today. (For example, the relatively low percentages for large planets are almost certainly due to the fact that these planets have long orbital periods, making most of them impossible for us to have detected during the short time in which we’ve been searching for them.)
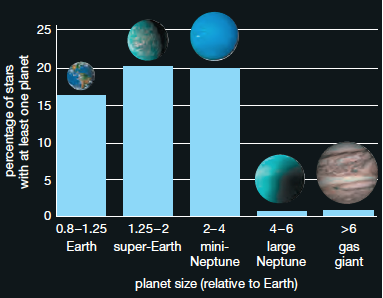
Figure 6. Estimated minimum proportions of all stars that have planets of different size categories, based on Kepler results. Image credit: The Cosmic Perspective, 8th Edition, by Bennett, Donahue, Schneider, and Voit (Pearson, 2017).
This brings us back to the question we opened with from St. Albertus Mangus. Although he could not have had the context we have today, we can guess that by “worlds,” he was probably thinking of worlds like Earth, with living beings. If so, then we still do not fully know the answer to his question, because we do not yet know whether any other worlds are home to life. But look how far we’ve come. There is no longer any doubt that worlds similar in size to Earth are common, and the fact that many of these worlds also have Earth-like orbits makes scientists suspect that worlds with continents and oceans are also common. With that, it seems only a matter of time until we discover worlds with life. If this occurs, as I expect it will, then we will have finally reached the culmination of the Copernican revolution: We will know not only that our Earth is not central to the physical universe, but also that it is not central to the biological universe.
For some, this likely-to-come discovery may be dismaying, seeming to diminish our own status in the universe. But I look at it differently: Using only the power of our bodies and brains, we, living here on one small world, will have figured out our true place in the universe. This is an achievement that should inspire anyone, regardless of whether beings on other worlds have done it before us. We have created something important here on Earth, and it is our job to make sure that we preserve it, so that our descendants can build upon it and someday set sail for planets around other stars.
About the Author
Jeffrey Bennett is the lead author of college textbooks in astronomy, astrobiology, mathematics, and statistics, as well as of numerous award-winning books for children and the general public. His personal web site is www.JeffreyBennett.com. Much of this article is adapted from material that appears the author’s textbook series from Pearson, written along with co-authors Megan Donahue, Nick Schneider, Mark Voit, and Seth Shostak. The series includes The Cosmic Perspective (8th edition), The Essential Cosmic Perspective (8th edition), The Cosmic Perspective — Fundamentals (2nd edition), and Life in the Universe (4th edition). More information can be found under the “textbook” section at http://www.bigkidscience.com/books/.